Contributed by Justin Miller, Bowman Lab
Alzheimer’s disease (AD), a dementia characterized by loss of memory and cognitive abilities, afflicts over an estimated 44 million people worldwide. While recent clinical trials have been exciting and suggest that slowing Alzheimer’s disease progression is possible, there are currently no FDA approved prophylactics or cures for Alzheimer’s disease. Given the lack of treatment options and the aging global population, the number of people impacted by Alzheimer’s disease is only expected to grow.
The strongest known risk factor for Alzheimer’s disease is the lipid transporter protein, apolipoprotein E, or apoE. Three variants of apoE are widespread in the global population and each carries a different risk for AD. apoE3 is the most common and carries a neutral risk, whereas carriers of the variant apoE4 are 12-15 fold more likely to develop AD. Carriers of the final variant, apoE2, are about half as likely as apoE3 carriers to develop AD, but these individuals often develop another disease, hyperlipoproteinemia. Recently, a fourth variant of apoE (apoE3 Christchurch) was reported where the patient described maintained high cognitive function despite numerous clinical markers of Alzheimer’s disease progression. Remarkably each variant of apoE is only a single amino acid different from apoE3. How exactly mutations in apoE contribute to AD, as well as how mutations in apoE impact the natural function of apoE, remains unclear. However, it is clear that apoE isoforms can impact AD, suggesting that therapies modulating the type of apoE an individual has may be a means of preventing or reversing AD progression. Our goal is to understand the different movements that apoE isoforms make and the different structures they tend to prefer with an end goal of better understanding how apoE contributes to AD. With this information in mind, we may be able to design drugs that correct the tendencies of apoE4 and make it mimic the less risky apoE3 or even the protective apoE2.
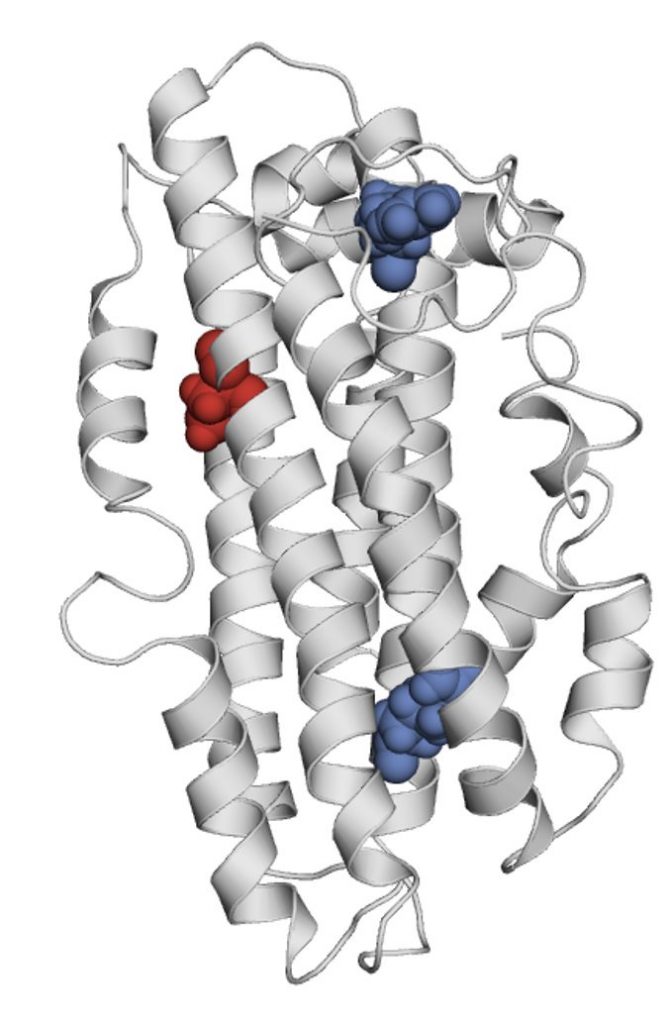
apoE has been difficult to study in the laboratory. Traditional experimental techniques used to study protein structures require high concentrations of protein where apoE may behave differently than in the body. Additionally, apoE has several regions of the protein that adopt more of a disordered conformation, which further complicates analysis via classic experimental methods. Molecular dynamics simulations, such as those employed by Folding@home are therefore uniquely suited for studying apoE. Computer simulations are robust at analyzing disordered proteins, and we can set up simulations such that a single apoE is being simulated at once. Because apoE is a highly flexible protein, we need to simulate apoE for a long time before we can make claims about the preferences of each apoE isoform. We are simulating each of the apoE isoforms mentioned above using GPU simulations in projects 18201 and 18202 and CPU based simulations in projects 18206 and 18210-18212. Once we have completed our simulations we will have accumulated over 65 Tb of simulation data for an aggregate simulation time of 63 milliseconds. Currently we have completed 15% of our data collection for this project. We are already seeing hints from our data that apoE explores more states than were previously expected.
We sincerely thank you for all of your contributions and eagerly await what this data can do for the Alzheimer’s disease community.